Glaucoma is a progressive optic neuropathy characterized by a loss of retinal ganglion cells (RGCs), leading to visual field defects and, ultimately, blindness. The pathophysiology of this disease remains incompletely understood. Although IOP is considered to be the most important and only modifiable risk factor in the development of glaucoma,1-3 RGC loss continues in some patients despite lowering of IOP, suggesting that other risk factors may contribute to neuronal loss in glaucoma.4,5 The mechanism of RGC apoptosis is likely multifactorial, with contributors including deprivation of neurotrophic factors, mitochondrial dysfunction, glial activation, excitotoxicity, failure of axonal transport, and oxidative stress (Figure 1).6,7
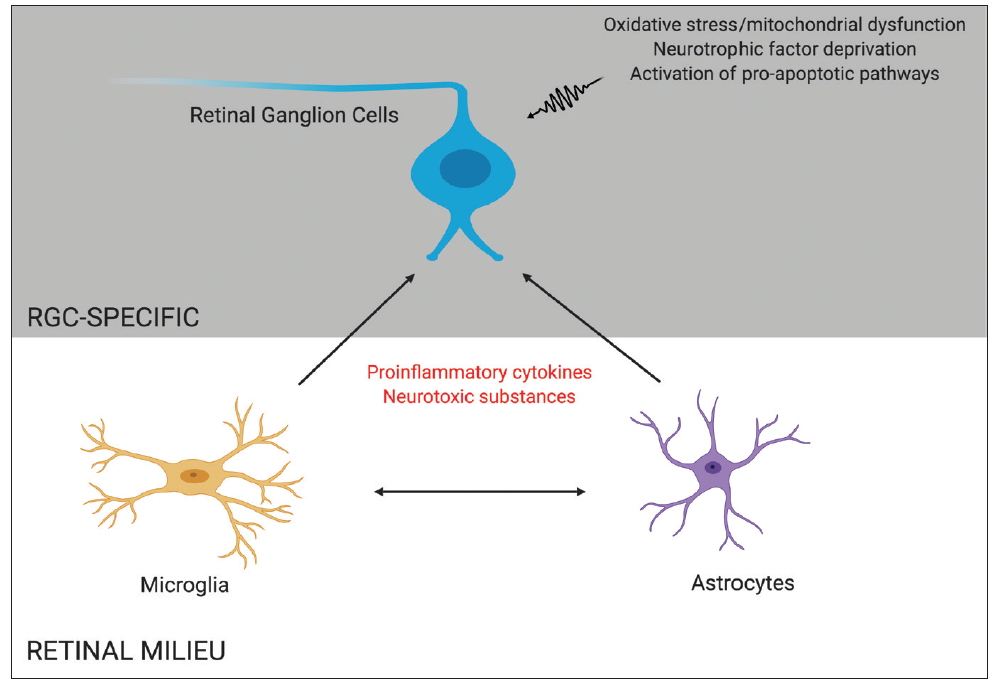
Figure 1. RGC death in glaucoma may be mediated by direct insults to RGCs, such as oxidative stress and neurotrophic factor deprivation, and by indirect proinflammatory effects caused by other retinal cells, such as microglia and astrocytes.
Neuroprotection in glaucoma is defined as an intervention that targets the molecular mechanisms causing RGC death and thereby enhances the survival of RGCs independent of IOP control. There is great interest in finding therapies that can offer neuroprotection in glaucoma. The course of research and development in this area has been rocky, but new approaches hold promise.
HISTORICAL APPROACHES
Investigators have tested many molecules for their neuroprotective effects in glaucoma, but success in their translation to clinical use has been limited. A case in point is memantine, a noncompetitive N-methyl-D-aspartate subtype of glutamate receptor antagonist. Already in use for treating Alzheimer disease, memantine showed early promise in protecting against RGC loss in animal models of glaucoma.8,9 A subsequent phase 3 randomized multicenter clinical trial, however, found that memantine treatment did not have a significant effect in preventing the progression of visual field loss in patients with glaucoma.10
A second example is brimonidine, a selective alpha-adrenergic receptor agonist approved for the long-term treatment of glaucoma. In a preclinical study, subcutaneous treatment with brimonidine improved RGC survival in an animal model of high IOP.11 Moreover, in the Low-Pressure Glaucoma Treatment Study (LOGTS), over a 30-month period, patients treated with brimonidine had a significantly lower rate of visual field defect progression compared to patients treated with timolol (9.1% vs 39.2%), although the mean treated IOP was similar for the two groups at all time points. The results of this study and the neuroprotective efficacy of brimonidine have since been questioned because of the higher rate of patient dropout, largely due to ocular allergies, in the brimonidine group (55% vs 29% in the brimonidine and timolol groups, respectively).12
Other molecules that have been tested for neuroprotective qualities in preclinical studies include brain-derived neurotrophic factor, human ciliary neurotrophic factor, calcium channel blockers, antioxidants, and ginkgo biloba extract.13 Further clinical studies are required to determine whether they have neuroprotective effects on glaucoma patients.
WHY SO MANY CHALLENGES?
There are many reasons why it is difficult to translate preclinical data into clinical practice. First, glaucoma is a heterogeneous disease that can be difficult to fully mimic in animal models. Human clinical trials include considerable disease variability, which can be caused by multiple factors, including medical comorbidities and polypharmacy.
Another fundamental difference between clinical trials and animal studies is the timing of intervention. In experimental studies, the neuroprotective agent is usually administered before or at the time of injury, whereas, in human clinical trials, patients are enrolled for intervention after being diagnosed with the disease.
There is also a difference in outcome measures between clinical and preclinical studies. In preclinical studies, histology is often employed to measure treatment efficacy. In contrast, in clinical trials, functional outcomes such as perimetry are frequently used, and these can take months to years to show change.
Lastly, it is often difficult to predict both the appropriate dosage of a neuroprotective agent for human use based on animal studies and the human ocular bioavailability.
NEW DRUG TARGETS
Focused on RGCs
Recent preclinical studies have focused on identifying cellular pathways that mediate RGC death to uncover new neuroprotective strategies. One study used an unbiased functional genomic screen of kinases involved in RGC death by delivering small interfering RNA to primary mouse RGCs.14 This and other work has identified dual leucine zipper kinase as playing an important role in RGC death in response to axonal injury, rendering dual leucine zipper kinase an attractive neuroprotective target.14-16 Subsequent high-throughput functional genomic screens have identified downstream signaling partners that may also be promising targets.17
Another study used RNA sequencing to identify molecular changes in RGCs that preceded glaucomatous neurodegeneration in a mouse model of age-related inherited glaucoma.18 The researchers showed that mitochondrial dysfunction occurred early in RGCs during glaucoma and that dietary supplementation of vitamin B3 and/or gene therapy driving expression of Nmnat1, a key enzyme producing NAD+, was protective against glaucoma.18
Clinical trials are needed to determine whether these interventions will benefit glaucoma patients.
Focused on Microglia and Astrocytes
The RGCs are contained within various cellular milieus, including the retina and the optic nerve head, which contain astrocytes, microglia and macrophages, and vasculature (Figure 2). Importantly, the survival of RGCs can depend on their interactions with these other cell types. Histopathologic studies of the glaucomatous optic nerve head have shown increased reactivity in astrocytes and microglia (resident macrophages in neural tissue), and these changes can have both protective and injurious effects on RGC survival.19 For example, in a mouse glaucoma model, ocular hypertension led to upregulation of the proinflammatory cytokine tumor necrosis factor (TNF)-alpha, followed by microglial activation and a delayed loss of RGCs.20 Inhibiting TNF-alpha through the systemic administration of etanercept has been shown to inhibit microglial reactivity and prevent axonal degeneration and loss of RGCs.21 By secreting TNF-alpha, other cytokines and complement proinflammatory microglia can in turn stimulate astrocytes to become directly and potently toxic to RGCs.22 These findings suggest that reducing proinflammatory cytokines and neurotoxic substances released by astrocytes and microglia may be viable neuroprotective strategies for glaucoma.
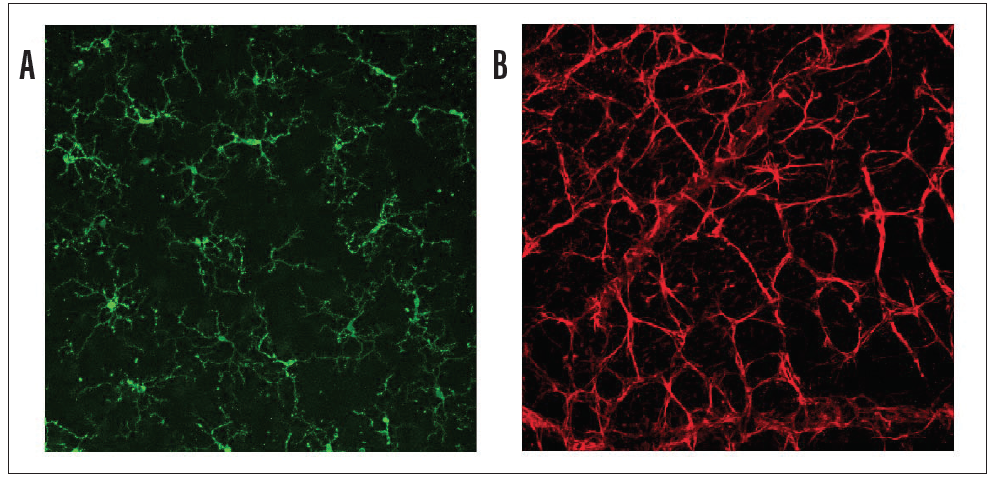
Figure 2. Mouse retina wholemounts showing retinal microglia stained with antibody against Tmem119 (panel A, green) and retinal astrocytes stained with antibody against glial fibrillary acidic protein (panel B, red).
GLAUCOMA CLINICAL TRIALS: NOVEL METHODOLOGIES
Use of novel methodologies and designs in clinical trials can aid translation of preclinical data to clinical use. For example, future clinical studies can incorporate the use of OCT in measuring peripapillary retinal nerve fiber layer thickness or macular ganglion cell complex thickness as outcome measures in addition to functional outcomes such as the visual field. Novel imaging techniques of identifying RGC death in vivo via direct detection of individual apoptotic retinal cells may enable high-throughput screening of candidate neuroprotective agents and provide more sensitive outcome measures for clinical trials.23,24
Although clinical trials thus far have failed to show any significant benefit of candidate neuroprotective agents in glaucoma, neuroprotection holds promise as a therapeutic strategy.
1. Gordon MO, Beiser JA, Brandt JD, et al. The Ocular Hypertension Treatment Study: baseline factors that predict the onset of primary open-angle glaucoma. Arch Ophthalmol. 2002;120(6):714-720; discussion 829-830.
2. Leske MC, Heijl A, Hyman L, Bengtsson B, Dong L, Yang Z; EMGT Group. Predictors of long-term progression in the Early Manifest Glaucoma Trial. Ophthalmology. 2007;114(11):1965-1972.
3. Quigley HA. Glaucoma. Lancet. 2011;377(9774):1367-1377.
4. Malihi M, Moura Filho ER, Hodge DO, Sit AJ. Long-term trends in glaucoma-related blindness in Olmsted County, Minnesota. Ophthalmology. 2014;121(1):134-141.
5. Peters D, Bengtsson B, Heijl A. Lifetime risk of blindness in open-angle glaucoma. Am J Ophthalmol. 2013;156(4):724-730.
6. Almasieh M, Wilson AM, Morquette B, Cueva Vargas JL, Di Polo A. The molecular basis of retinal ganglion cell death in glaucoma. Prog Retin Eye Res. 2012;31(2):152-181.
7. Qu J, Wang D, Grosskreutz CL. Mechanisms of retinal ganglion cell injury and defense in glaucoma. Exp Eye Res. 2010;91(1):48-53.
8. Hare WA, WoldeMussie E, Weinreb RN, et al. Efficacy and safety of memantine treatment for reduction of changes associated with experimental glaucoma in monkey, II: structural measures. Invest Ophthalmol Vis Sci. 2004;45(8):2640-2651.
9. Lagrèze WA, Knörle R, Bach M, Feuerstein TJ. Memantine is neuroprotective in a rat model of pressure-induced retinal ischemia. Invest Ophthalmol Vis Sci. 1998;39(6):1063-1066.
10. Weinreb RN, Liebmann JM, Cioffi GA, et al. Oral memantine for the treatment of glaucoma: design and results of 2 randomized, placebo-controlled, phase 3 studies. Ophthalmology. 2018;125(12):1874-1885.
11. Lambert WS, Ruiz L, Crish SD, Wheeler LA, Calkins DJ. Brimonidine prevents axonal and somatic degeneration of retinal ganglion cell neurons. Mol Neurodegener. 2011;6(1):4.
12. Sena DF, Lindsley K. Neuroprotection for treatment of glaucoma in adults. Cochrane Database Syst Rev. 2017;1:CD006539.
13. Doozandeh A, Yazdani S. Neuroprotection in glaucoma. J Ophthalmic Vis Res. 2016;11(2):209-220.
14. Welsbie DS, Yang Z, Ge Y, et al. Functional genomic screening identifies dual leucine zipper kinase as a key mediator of retinal ganglion cell death. Proc Natl Acad Sci U S A. 2013;110(10):4045-4050.
15. Fernandes KA, Harder JM, John SW, Shrager P, Libby RT. DLK-dependent signaling is important for somal but not axonal degeneration of retinal ganglion cells following axonal injury. Neurobiol Dis. 2014;69:108-116.
16. Watkins TA, Wang B, Huntwork-Rodriguez S, et al. DLK initiates a transcriptional program that couples apoptotic and regenerative responses to axonal injury. Proc Natl Acad Sci U S A. 2013;110(10):4039-4044.
17. Welsbie DS, Mitchell KL, Jaskula-Ranga V, et al. Enhanced functional genomic screening identifies novel mediators of dual leucine zipper kinase-dependent injury signaling in neurons. Neuron. 2017;94(6):1142-1154.e6.
18. Williams PA, Harder JM, Foxworth NE, et al. Vitamin B3 modulates mitochondrial vulnerability and prevents glaucoma in aged mice. Science. 2017;355(6326):756-760.
19. Chong RS, Martin KR. Glial cell interactions and glaucoma. Curr Opin Ophthalmol. 2015;26(2):73-77.
20. Nakazawa T, Nakazawa C, Matsubara A, et al. Tumor necrosis factor-alpha mediates oligodendrocyte death and delayed retinal ganglion cell loss in a mouse model of glaucoma. J Neurosci. 2006;26(49):12633-12641.
21. Roh M, Zhang Y, Murakami Y, et al. Etanercept, a widely used inhibitor of tumor necrosis factor-α (TNF-α), prevents retinal ganglion cell loss in a rat model of glaucoma. PLoS One. 2012;7(7):e40065.
22. Liddelow SA, Guttenplan KA, Clarke LE, et al. Neurotoxic reactive astrocytes are induced by activated microglia. Nature. 2017;541(7638):481-487.
23. Yang E, Al-Mugheiry TS, Normando EM, Cordeiro MF. Real-time imaging of retinal cell apoptosis by confocal scanning laser ophthalmoscopy and its role in glaucoma. Front Neurol. 2018;9:338.
24. Liu WW, Margeta MM. Imaging retinal ganglion cell death and dysfunction in glaucoma. Int Ophthalmol Clin. 2019;59(4):41-54.